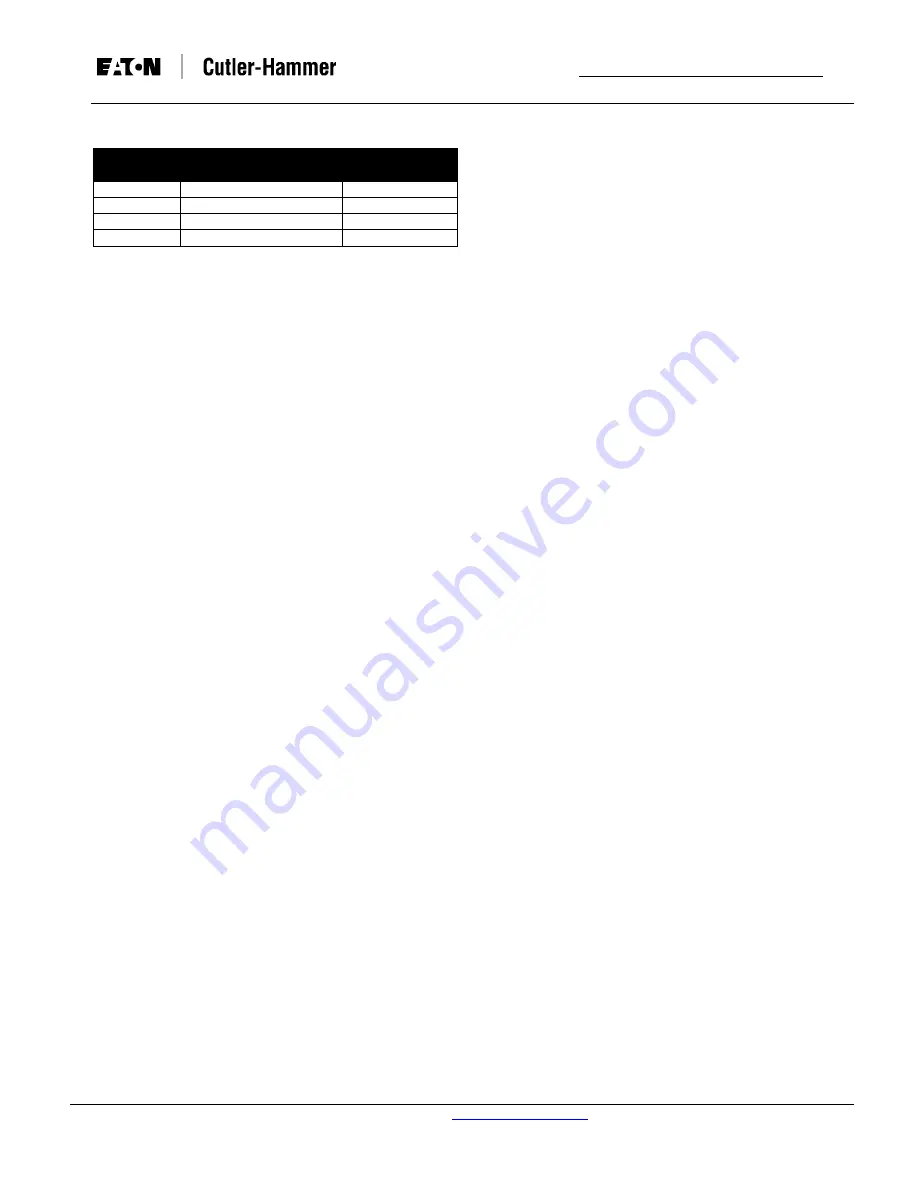
DT3000
Instruction Leaflet
I.B. 17555D
Effective: Date 07/02
Page 21
For more information visit:
www.cutler-hammer.eaton.com
Supersedes I.B. 17555C dated November 1999
TABLE 3.1 DIGITRIP 3000 CURVE SHAPES
Thermal
Curves
ANSI Curves
(per ANSI C37.112)
IEC Curves
(per IEC 255-3)
It
Moderately Inverse
IEC-A
I
2
t
Very Inverse
IEC-B
I
4
t
Extremely Inverse
IEC-C
FLAT
IEC-D
As shown in Figure 3-1, the ANSI and IEC “Curve
Shapes” are in terms of multiples of (Pickup Current
of the Ct Primary), whereas ‘short delay” and
“instantaneous” are in terms of multiples of I
n
(5A
secondary of Ct primary current). The thermal curve is
represented in terms of multiples of I
n
for its curve
shape, short delay, and instantaneous settings. This
must be considered in the coordination study and in
the programming of the Digitrip 3000 Protective Relay.
ANSI C37.l 12 defines the ANSI curves and IEC curves
are defined by lEC 255-3. These curve shapes combine
with the customized capability of the short delay and
instantaneous functions to allow for very versatile
coordinated protection schemes. The user can also
customize the thermal curve shape to any desired type of
coordinated protection scheme.
These curves show how and when a particular relay will
act for given values of time and current. Because the
DT3000 is very versatile the easier it is to accomplish
close coordination and achieve optimum protection. The
makeup of a typical curve is presented for clarification
purposes.
For the sake of simplification, the curve discussion will
center on a single line curve. Keep in mind, however, that
a characteristic curve in reality is represented by a band of
minimum and maximum values, not a line (Figure 3-1).
Minimum and maximum values are generally the result of
tolerances introduced by the manufacturing process for
components and the relay’s accuracy. Any expected value
of tripping current or time could be the nominal value
anticipated within the plus or minus tolerance. The
tolerances just mentioned are usually stated in terms of the
relay’s accuracy and frequently highlighted on the actual
working curves. Accuracy is stated in terms of a plus or
minus percentage and represents a permitted fluctuation
on either side of the nominal tripping point for a family of
relays, like the Digitrip 3000.
The ability to adjust the relay and the continuous current of
the Digitrip 3000 Protective Relay are two factors that
contribute significantly to the great flexibility.
a)
Adjustable:
The ability to adjust the relay permits
movement of its characteristic curve or parts of the
curve. This movement can be done in both a
horizontal and vertical direction on the time current
grid. The actual shape of the curve can be changed
along with the curve movement. This ability permits
distinct curves to be established that will better match
the electrical protection to the application need
(Figures 3-3 through 3-9) Notice that there is no
horizontal movement of the ANSI and IEC curve
shapes. Only the point at which the relay starts to time
out moves along the curve shape.
b)
Nominal Continuous Current:
The Digitrip 3000’s
nominal continuous primary current (I
n
) is established
by the ratio of the selected current transformers. The
current transformer ratio must be set via the initial
programming of the relay. These settings must agree
with the circuit current transformers to which the relay
is connected. Therefore, I
n
is established by the
current transformer ratio used and becomes the
primary scale factor for the trip functions and
readouts.
Before proceeding with the curve explanation, it should be
noted that combining functional capabilities, such as
inverse time overcurrent, short delay and instantaneous, is
a coordination activity. The effects of one set of settings on
another setting should always be evaluated to determine if
the results under all possible circumstances are
acceptable. This helps to avoid unexpected operations or
non-operations in the future. Such possibilities are
highlighted at the end of this discussion as a reminder
when establishing relay characteristic parameters.
Inverse Time Overcurrent Protection
Inverse time overcurrent protection consists of a curve
shape pickup setting and an inverse time multiplier setting.
The inverse time overcurrent function offers eleven
possible curve shape types as previously described
(Figure 3-2 and Table 3.1). When programming the relay,
this will be the first choice to make. The curve shape and
its effect on the characteristic curve will be covered with
the time multiplier explanations.
The pickup setting establishes the current level pickup at
which the relay’s inverse time overcurrent tripping function
begins timing. If, after a predetermined amount of time, the
current condition that started the timing process still exists,
the relay’s trip relay is energized. Pickup settings can be
adjusted from 0.20 to 2.20 times I
n
for the standard
DT3000.
The Pickup settings for the Chicago version
(DT3100 and DT3101) can be adjusted from 0.20 to 1.00
times I
n.
Refer to Tables 2.2 and 2.3 for a complete list of
available settings. Figure 3-4 graphically illustrates how the
Inverse Time Overcurrent Pickup portion of the overall
curve can be moved horizontally on the time current grid
by means of the pick-up settings. The Inverse Time
Overcurrent Pickup is represented by the dotted lines,
while a solid line represents the rest of the curve.
The Time Multiplier setting is used to select a
predetermined amount of time a sustained overload
condition will be carried before the breaker trips. For the
Thermal Curves, a value of (3 x I
n
) is the reference point
where the programmed time multiplier setting is fixed on
the curve. Wide ranges of time settings are available and
depend upon the curve shape selection. As Time Multiplier
settings are varied, the Time Multiplier portion of the
overall curve is moved vertically up or down on the time
current grid. This movement is also independent of the