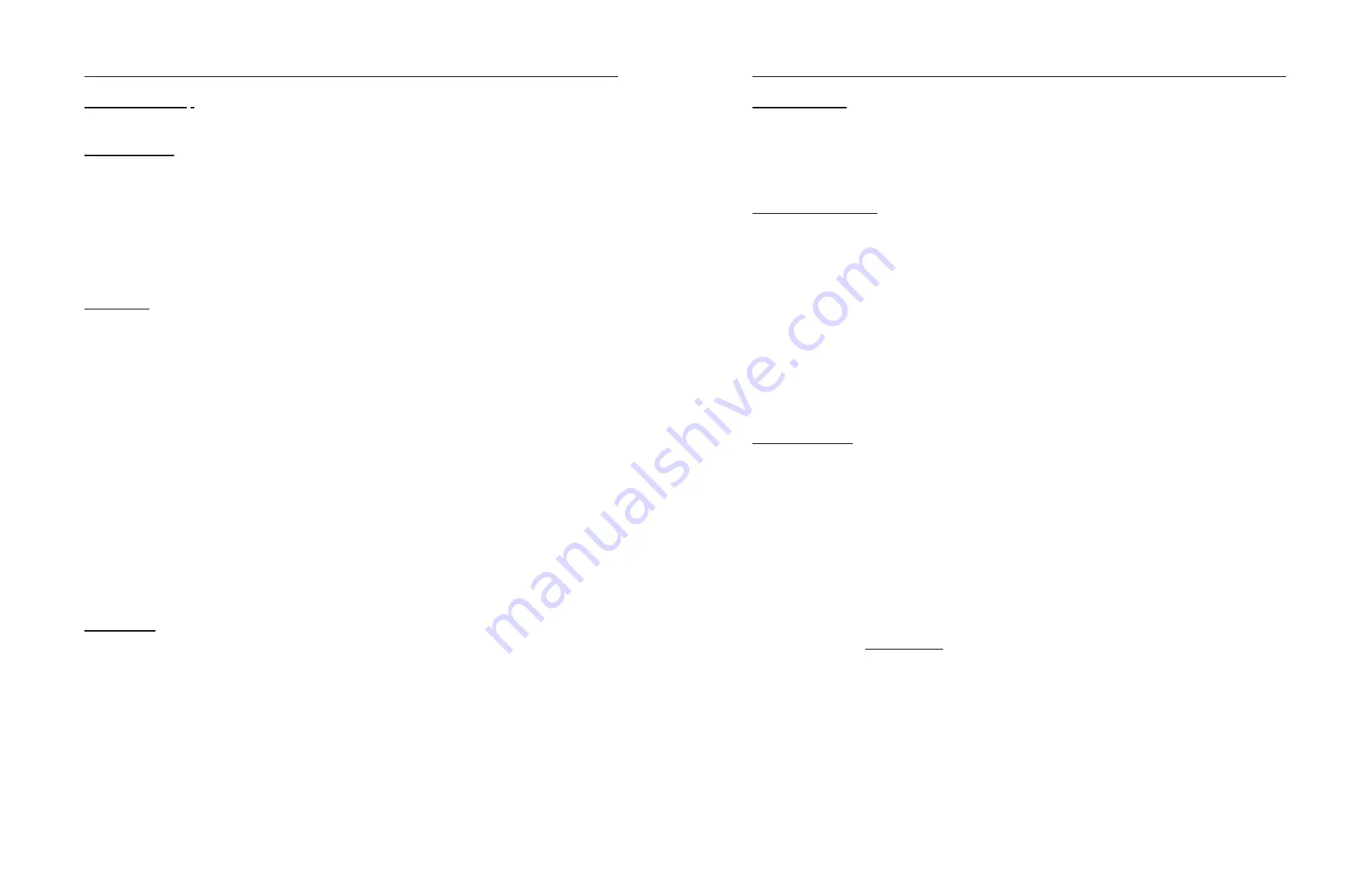
SECTION 15 FINAL INSPECTION AND FLIGHT TEST
RV AIRCRAFT
15-11
SEC 15r8 12/23/10
Best Angle Of Climb:
After climb tests have been made at normal anticipated speeds, perform timed climbs at indi-
cated speeds of 90 IAS, decreasing in 10 mph increments, down to 70 mph. At the lower speeds, watch cylinder
head and oil temperatures carefully to avoid overheating. Limit low airspeed climbs to 2 minutes or less for cooling
reasons.
Plot Climb Charts:
Following the procedure outlined here, plot on graph paper a climb curve for various airspeeds.
After the points have been located for climb rates at different speeds, draw in a smooth curve which connects all
points. Because of testing inaccuracies, the climb curve can be drawn to a smooth shape which approximately con-
tacts the points. The speed for maximum climb rate is that at the top of the curve. As the speed increases past that
point, the climb rate will decrease until it is zero at the airplane's top level flight speed.
Draw a straight line from the 0-0 beginning point of the chart up to a point where it is tangent to the curve. This point
will be the BEST ANGLE OF CLIMB SPEED.
Plot another curve of climb rate vs. altitude for the best climb rates for each altitude. The best rate of climb will of
course be at the lowest altitude, and will decrease with increasing altitude. A line connecting these points will be a
straight line, and an upward projection of this line will provide the theoretical absolute ceiling of the airplane. The ser-
vice ceiling would be the altitude at which a 100 fpm climb rate is indicated. On the other end of the line, where it
intersects the “0” altitude line, will be the SEA LEVEL climb rate. Using these graphs, reasonably accurate ceilings
and sea level climb rates can be found without ever flying at those exact conditions.
Slow Flight:
The idea is to become familiar with the trim and attitude changes that take place while you are trying to
maintain your altitude at minimum flight speed. Careful, you could stall unexpectedly. Do these maneuvers at a safe
altitude. Try a few level turns with and without flaps. But, do a lot of slow flight practice. Practice until you can con-
sistently maintain speeds within 5 mph above stall speed while transitioning from wings level through 15-20 degree
banks to the right and left. The idea is to become so familiar with slow flight that it can be done almost subcon-
sciously; being able to devote thought to traffic and other considerations at the same time. You want to be very famil-
iar with the slow flight mode so that you are able to make landing approaches safely even with the distractions which
are bound to occur, and to be able to detect the approach of stall speed even while dividing your attention to other
factors such as traffic and ground obstructions.
However, watch your engine temperatures while practicing slow flight. The reduced cooling airflow, coupled with the
relatively high power required for slow flight, will cause the engine to heat more than at cruise conditions. Slow flight
practice sessions will probably have to be limited in duration for this reasons.
Years of experience gained from reviewing accidents and flight control problems have shown that a better mastery of
slow flight could have prevented many accidents and minimized flight difficulty problems. ABE accident statistics
show that 18% of accidents occur on takeoff and 33% on landing. Both of these flight regimes involve slow speed
flight and the need for control under these conditions.
Takeoff: There are a few critical seconds following takeoff where flight must be controlled within a few mph of stall
speed and where wind turbulence can significantly affect attitude, controllability, and airspeed. Even further into the
takeoff/departure sequence, climb speeds can deteriorate to critically low margins because of turbulence, wind shear,
obstacle clearance requirements, and other distractions.
Landing: The historical evidence of landing approach stall/spin accidents should be sufficient evidence of the need
for a high level of pilot familiarity with low speed controllability. Slow flight skills are also of great importance to the
final phase of the landing; pre-touch down. Particularly for tail wheel airplanes making full flare, 3 point landings, the
last few seconds preceding touch down are crucial. Look at it this way. The pilot must keep the airplane under con-
trol within a few feet of the runway, within a few mph of stall speed, and in a straight line relative to the surface. Are
these the circumstances under which slow flight should be learned? Of course not! Learn at altitude when time and
distance(altitude) are in your favor. Then as you approach the critical landing sequence, many of the needed skills
will have already been acquired.
Gliding Tests:
In the event of an engine failure, it would be nice to know what airspeed will give the minimum gliding
angle. These tests, logically, are most effectively performed following your climb tests because you could then use
the altitude gained.
Start with plenty of altitude and complete your last practice turn at least 1000' AGL. Clear your engine briefly after
each 90 degree turn. If you don't have a VSI, time your descent through different thousand foot levels.
To learn how your airplane behaves in gliding turns practice a few and note how the rates of descent change with
airspeed and bank angle. It is important to keep your gliding turns coordinated. Try doing them at different airspeeds
and record your observations.
Practicing these gliding turns is essential because you will be duplicating them each time you turn final for landing.
Be careful -- an excess of uncoordinated rudder input (slip or skid) and excessive back pressure on the stick can
cause the airplane to snap over the top, or snap under to an inverted attitude. At traffic pattern altitude, this can be
fatal.
Determine and record how much altitude is ordinarily lost in making a 90 degree gliding turn, a 180 degree and a 360
degree turn. Make similar checks with partial and full flaps.
SECTION 15 FINAL INSPECTION AND FLIGHT TEST
RV AIRCRAFT
15-12
SEC 15r8 12/23/10
Plot Glide Speeds:
On the same graph as climb performance, plot points for gliding rates of descent (sink) at vari-
ous speeds tested. In addition to the rates of sink listed for the various speeds, a tangent line can be drawn to find
the speed at which the best glide angle can be attained. By converting MPH to FPM forward speed, and then divid-
ing by the sink rate in FPM, the glide ratio can be found.
While these sink rates and speeds are valuable guidelines, they are not totally representative of those which might be
experienced during an actual engine failure emergency. At idle power, a fixed pitch RV will show a better glide ratio
and angle than it will at zero power and the prop windmilling. The glide ratio with the prop windmilling (no combus-
tion) will be better with the throttle open than if it were closed. The bottom line is that the pilot should be able to visu-
ally access the glide performance on the spot and plan his power off approach accordingly.
Engine Cooling Checks:
Monitor and record engine temperatures on every flight. However, you should also study
and record the effects produced by aggressive mixture control manipulation, changes in airspeeds, and changes in
power setting. Prolonged climbs and glides will probably produce dramatic changes in engine temperatures and you
should know to what degree. Remember, hot summer free air temperatures can intensify high engine temperature
indications - often to a critical degree.
STABILITY INVESTIGATIONS
One of the more subjective areas of flight testing is that of aircraft stability. It is necessary to check for stability in all
three axes, LONGITUDINAL (pitch), LATERAL (roll), and DIRECTIONAL (yaw.) Stability testing cannot be accom-
plished until the airplane has been checked for trim and any external tabs needed have been installed and adjusted
to permit control free (hands off) flight. Before describing how to perform stability checks, we’ll first define what the
various forms of stability are.
x
Longitudinal (Pitch) Stability
: The tendency to remain at a constant trim speed, and to return to a that trim speed
after being displaced by a pitch control input.
x
Lateral (Roll) Stability:
The tendency of the bank angle to remain constant, or to return to wings level.
x
Directional (Yaw) Stability:
The tendency of an airplane to maintain a directional heading when wings are level
(no roll), and to return to a steady heading after release of a yaw input control (rudder).
Before describing the testing procedure, lets review some theory:
C.G. Considerations
: While performing stability checks, it is important to that the pilot recognize the effects of the
position of the C.G.
Pilots have all been exposed to the term "aft center of gravity" and are aware that this is a condition which has limits
and is normally referred to in a precautionary tone. But, how well do you understand all of the ramifications of aft
C.G. conditions? Perhaps you know that this is a condition to be avoided when doing aerobatics, but do you know
how to recognize the symptoms of aft C.G. in normal flight conditions, or the problems which may be encountered
under "normal" conditions as a result of an aft C.G. condition?
Fig. 15-1 shows the basic forces acting on an airplane. This airplane is designed to have positive stability with the
C.G. located as shown. It is in equilibrium at a design cruise speed. The nose down tendency caused by the C.G.
being forward of the center of lift (C.L) is balanced by the stabilizer down load resulting from the negative incidence
angle (relative to the wing angle) of the stabilizer. So, a constant static load is balanced by an aerodynamic force
which will vary with airspeed. If the aircraft's nose is lowered, an increase in speed will result, and that will cause a
greater down-load on the stabilizer, which will in turn raise the nose again to bring the speed back to where it started.
The converse will happen if the nose is raised. However, the aircraft will normally overshoot its original trimmed atti-
tude and speed. Thus, there are usually several cycles of pitch hunting required to return to stable flight. Each cycle
is of decreasing amplitude (altitude variation). These pitch cycles are called “phugoids”.
Fig. 15-2 shows the aircraft loaded to a more forward C.G. condition, for which an elevator trim force is needed to
maintain equilibrium. Generally, a nose heavy airplane is more stable because of the greater difference between the
static weight position and the dynamic force of the trimmed elevator.
Fig. 15-3 shows the aircraft loaded so the C.G. and the Center of Lift are at the same point. Thus, no stabilizing trim
load would be required of the tail. But, if there is no trim load, there no restoring load, and thus no positive stability.
In this condition the aircraft would have neutral stability. It would continue to fly at whatever attitude it is placed or
displaced to.
Fig. 15-4 shows the aircraft loaded to an extreme where the C.G. is aft of the Center of Lift, and where the horizontal
tail surfaces must produce a lift force to maintain level flight. In this condition, when the nose is lowered, speed will
increase and the stabilizer force will increase. But, since it is a lifting force or upload on the tail, it will continue to
lower the nose and produce more lift and more speed. etc. If the nose were raised and the speed decreased to below
trim speed, the reverse would occur; speed would continue to drop until a stall occurred, recovery from which would
be difficult and spin entry would be probable. This is an unstable condition because the forces acting on the aircraft
are destabilizing with a change in speed. In this condition the aircraft is PITCH DIVERGENT and is extremely difficult
to fly and dangerous.